MAAVi Innovation Center
15 years obsessed
with the power of nature
WE BELIEVE IN A DIFFERENT WAY OF PRODUCING FOOD
We leverage on the knowledge accumulated by nature over millions of years of evolution, boosting it with our technology and with Artificial Intelligence.
We understand nature, decode it, and maximize its potential. We call it Natural Intelligence (NI)
MAAVi Innovation Center, Europe’s largest hub of biotechnological innovation applied to the study of natural molecules obtained from botany, microbiology, microalgae and bioinformatics. We have 15 years of expertise researching natural compounds in agriculture, aquaculture, animal production, the food industry, cosmetics, and other sectors interested in transitioning to a natural model.
What was our motivation?
We saw the need for better, more sustainable food production long before most. As a consequence, we research to get natural solutions tailored to specific needs, assuring efficacy equivalent to akin chemicals in a wide range of sectors.
Given the regulatory changes in Europe and the growing demands of distributors and consumers worldwide, we strive to keep the industries in which we operate profitable and productive.
Our objective is to accelerate the transition from synthetic to natural chemistry.
We believe in a new way of engaging with the environment that is based on real sustainability on all levels, including economic, social, and environmental sustainability.
Because nature has all of the solutions; all we have to do is find and use them.
environment
+10.000
square meters dedicated to R+D+i
global
+1.800
associated agronomists worldwide
neurons
+80
top-level scientists from numerous specialized fields
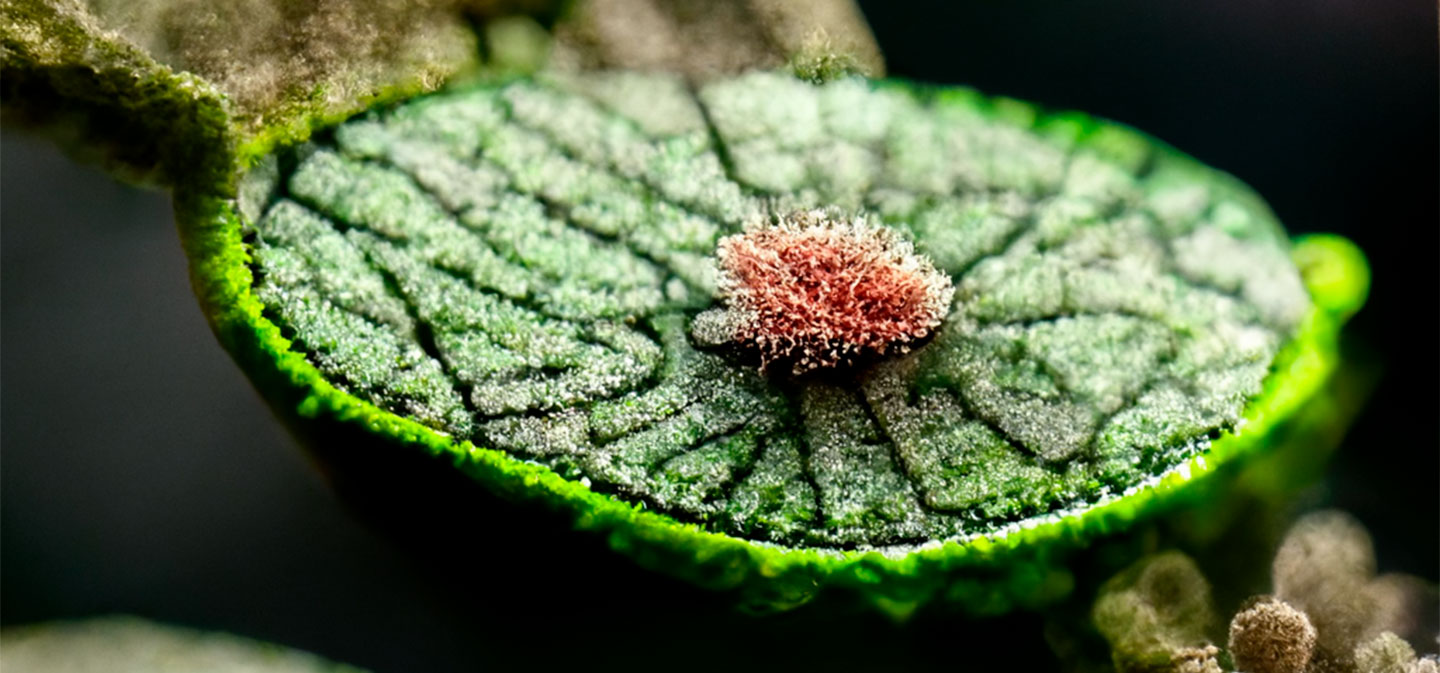
Who we aren’t
- We are not a conventional research center
- We are not an agrochemical multinational
- We are not your typical natural company
We are neither conformists, conservatives, or a grey company.
We are unique; we break established rules and change the way that things are done.
We are nonconformists by nature, obsessed with the pursuit of excellence.
Still in doubt? keep reading!
We are not a conventional research center.
We will not be happy until we have found your solution on an industrial scale.
From pest control to the development of a new molecule that improves the functionality of a product
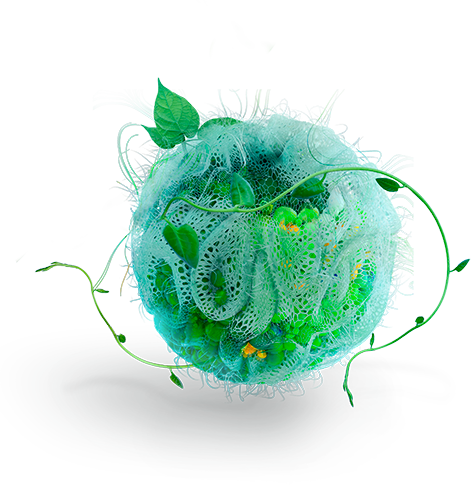
Basic Science

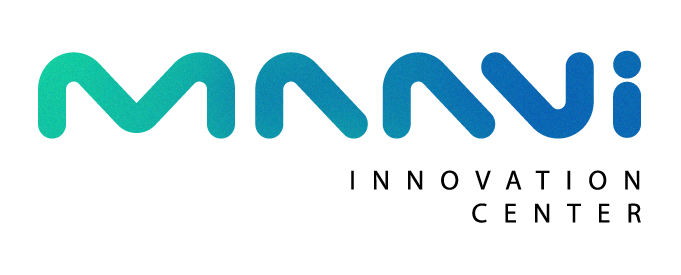

We are not your typical natural company.
We base our work on a pharmacological, effective, and accurate model .
Unlike other natural companies, we do not speak in terms of extracts, but rather about natural compounds. Natural compounds that we identify and analyze in all of their complexities in order to understand patterns, as well as the synergies and interactions between them, in order to maximize their potential.
- Our working methodology (LRP) makes us unique because it comprises a natural matrix-based selection and amplification mechanism.
- Because we guarantee the efficacy of natural compounds with activity through research.
- Because we understand and harness the potential of synergistic interactions between molecules in complex matrices.
As in pharma, efficacy and homogeneity are required to provide consistent outcomes.
MAAVi Innovation Center is a high-performance biotechnology innovation research center.
Here is how we do it
1.
WE START FROM A NEED
We attend to and understand a specific market challenge.
2.
AMPLIFICATION: WE ARE AWARE OF ITS SCOPE
We are aware of its global influence thanks to our Business Intelligence team.
3.
FIRST, INFORMATION
We analyze the problem and rely on hundreds of professionals to comprehend it from the market’s perspective.
4.
WE START R+D
After understanding the problem’s dimensions and using a 360º approach, we proceed in the laboratory
5.
WE FACE THE CHALLENGE
We perform hundreds of in silico, in vitro, in vivo, and on site tests and trials. We validate Hits, Leads, synergies and prototypes. We then proceed to scaling and registration.
6.
WE MAKE IT HAPPEN
We manufacture and distribute the finished product.
We are not your typical natural company.
We never utilize anything that isn’t found in nature.
All of the compounds we use come from nature, which has evolved over millions of years to have the mechanisms necessary to properly break them down. As a result, they do not qualify as POPs or PBTs since they are not bioaccumulative, persistent, or toxic, and because they are natural compounds, they are biodegradable. They are extracted directly from nature through biological processes. We guarantee homogeneous results, the same efficiency as synthetic chemicals, and a reduction in the appearance of resistance.
We speak your language
BACK TO END Or in other words, from the need to the final solution. Agriculture, the food industry, and other sectors are currently undergoing significant changes that need thorough, swift, and efficient frameworks. MAAVi Innovation Center is your industrial and R+D partner. You are unique, but so are we.
Efficacy
See more
Our solutions guarantee efficacy equal to or greater than that of the market’s reference synthetic chemistry products.
Competitiveness
See more
Going natural should not imply paying more. We work to improve the competitiveness of our products. We cannot be an alternative if we are not competitive.
Scalability
See more
We guarantee the scalability of our processes, ensuring homogeneity and efficacy.
Registrability
See more
Natural does not mean harmless. We analyze natural compounds and take steps to ensure food safety.
Manufacturing
See more
We have a manufacturing line with a capacity of 120 million liters per year in a facility equipped with 4.0 technology.
Europe’s largest biotechnology innovation hub at your service
Growers
Are your crops being attacked by pests for which there is no available treatment? Are you worried about the impact of climate change on your crops? Are you aware that multinational companies overlook minor pests?
See more
- We implement a strategic plan designed to answer all your needs.
- We assist you in achieving zero residue in crops by effectively combating pests and diseases.
- We maintain your profitability and productivity.
- We help you in tackling the challenge of climate change adaptation.
- We help you respond to the demands of both consumers and distribution chains, as well as regulatory changes.
Through strategic alliances, we put the research potential of our High-Performance Center at your service in order to provide you with tailored solutions to your challenges in the form of a product. We are your current and future R&D&I partner, committed to guarantee your economic sustainability.
Food industry
he agroindustry has three primary challenges: limiting chemical transmission to raw materials, replacing chemical additives for natural substances (substituting E numbers), and achieving sustainability through reduced environmental impact.
See more
The time for highly effective natural compounds has come. Our 15 years of experience make us the perfect strategic partner for agrochemical companies willing to accelerate their transformation without jeopardizing their profitability.
We can provide them with the answers they need in the form of a highly effective, alternative product that can substitute specific molecules, ensuring the profitability of their portfolio.
Competitive solutions suitable for registration and fully scalable, to make the transition from synthetic chemicals to natural inputs REAL and FAST.
Agrochemical industry
Welcome to the natural
revolution in food production.
The world is changing and, although agrochemical companies and their tools based on synthetic chemistry have been key to increase productivity in food production, people are now demanding a change towards alternative technologies, such as biostimulants and biopesticides, which are equally effective and homogeneous, but more respectful to the environment and human health. It is now the time to go natural instead of eco-friendly!
See more
The industry is facing major changes at breakneck speed. Given the necessity to transform the model, our Open Innovation 360 offers optimal solution for the entire supply chain.
We propose circular economy-based approaches for revaluing byproducts.
See more
- We implement a strategic plan designed to answer all your needs.
- We assist you in achieving zero residue in crops by effectively combating pests and diseases.
- We maintain your profitability and productivity.
- We help you in tackling the challenge of climate change adaptation.
- We help you respond to the demands of both consumers and distribution chains, as well as regulatory changes.
Through strategic alliances, we put the research potential of our High-Performance Center at your service in order to provide you with tailored solutions to your challenges in the form of a product. We are your current and future R&D&I partner, committed to guarantee your economic sustainability.
See more
The time for highly effective natural compounds has come. Our 15 years of experience make us the perfect strategic partner for agrochemical companies willing to accelerate their transformation without jeopardizing their profitability.
We can provide them with the answers they need in the form of a highly effective, alternative product that can substitute specific molecules, ensuring the profitability of their portfolio.
Competitive solutions suitable for registration and fully scalable, to make the transition from synthetic chemicals to natural inputs REAL and FAST.
See more
The industry is facing major changes at breakneck speed. Given the necessity to transform the model, our Open Innovation 360 offers optimal solution for the entire supply chain.
We propose circular economy-based approaches for revaluing byproducts.
She is wise. She is complex. She is our ally.
The chemical spectrum of natural products is enormous (billions of compounds) and contains unlimited combinations of molecular structures found in nature.
Only around 1% of that chemical variety is known, with plants, terrestrial microbes, and marine creatures accounting for more than 80%. Current databases contain between 300,000 and 500,000 compounds, with an average of 3000 molecules reported every year, the vast majority of which have no known biological function.
Nature is our most valuable source of information, but most of it is hidden. Molecules and their interactions include biological characteristics. Specific functions are encoded inside the molecular structure. Decoding nature, revealing its secrets and applying its wisdom are our biggest challenges.
Can you imagine the possibilities?
Nature is our most valuable source of information. Our task is to decode and illuminate its hidden aspect.
We rely on human and technology resources, and artificial intelligence serves as an amplifier and booster of our natural intelligence expertise.
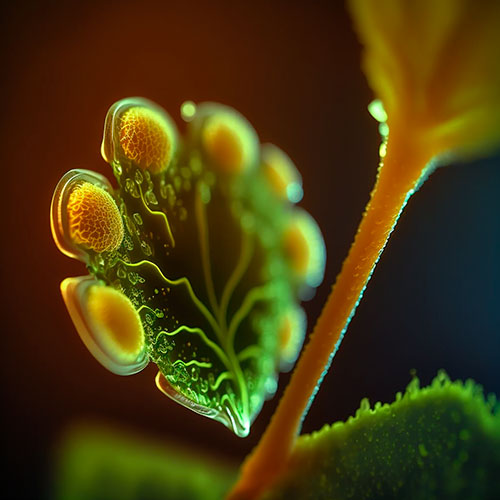
Sources of natural compounds
We capitalize the knowledge accumulated over millions of years of natural evolution, studying nature’s ways, shedding some light on its hidden compounds and gaining access to its riches through biotechnological processes.
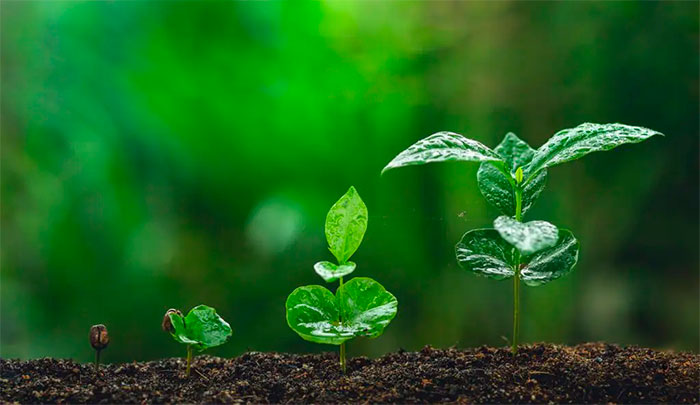
Botany
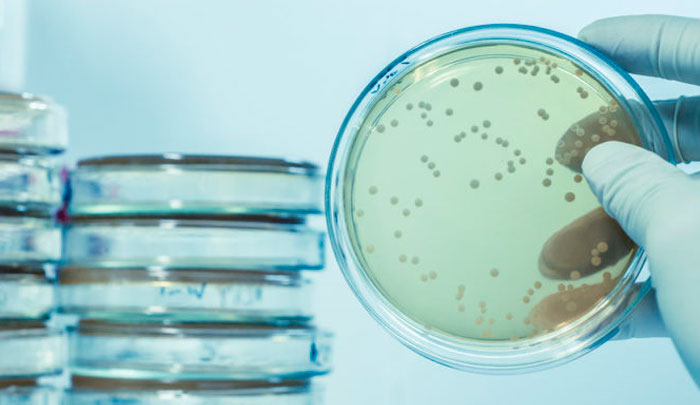
Microbiology
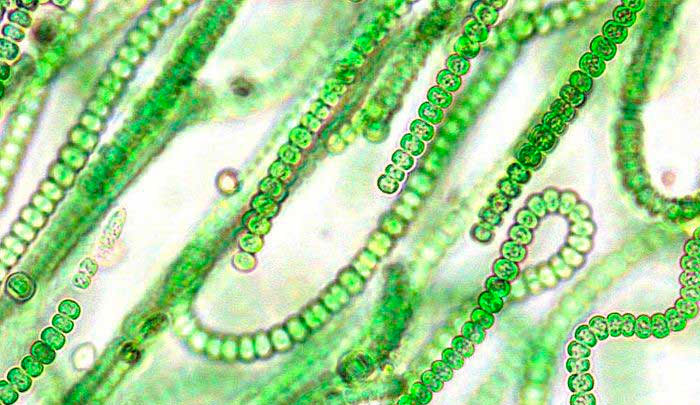
Microalgae
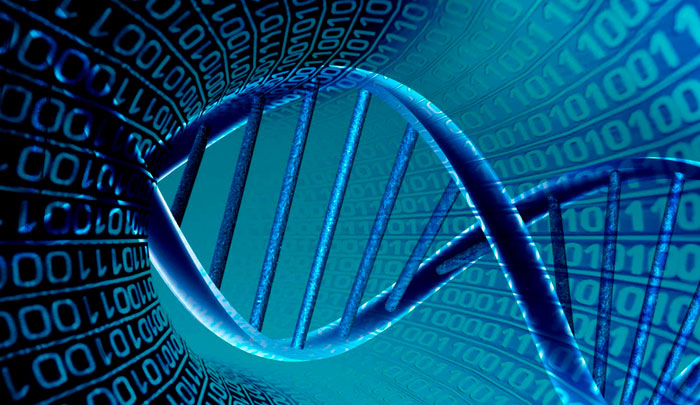
Bioinformatics
Learn about our research areas
AND THE OSCAR OF SCIENCE GOES TO…
We are the only biotech company with 5 projects of the European Union R&D framework program
We are leaders in the field of scientific research and innovation in Europe. We are the only biotech company with 5 Horizon projects, the first funding program of the European Union for research and innovation purposes. A recognition of scientific excellence.
First and only horizon 2020 project in biopesticides
Until 2015, the EU showed no interest in biopesticides. That year, Europe launches a financed research line in this field, and called it Horizon 2020. Among hundreds of participants, Europe determined that Kimitec, the MAAVI Innovation Center’s founding company, was the best choice to lead the industry. They chose us, and it was the right choice. That year, we were granted the first and only Horizon 2020 project on biopesticides, which eventually became the basis of our current biopesticide line.
The project was named BLOSTER, and it was the first time that Europe endorsed our vision on the importance of researching and bringing to market actual alternatives to synthetic chemicals, replacing harmful molecules to human health and the environment with those obtained from natural sources. That is how we obtain completely natural biopesticides that are just as effective as synthetic chemicals and offer the highest level of phytosanitary safety.
Economic impact, high quality and real sustainability, in contrast with conventional pesticides.
4 HORIZON 2020 / 1 HORIZON EUROPE
Learn about all our European projects
We have participated in more than 25 European funded projects aimed at transforming the food production system by eliminating synthetic chemicals and favoring fully natural products.
Further information on our projects
BLOSTER
Did you know that the EU showed no interest in biopesticides until 2015? That year, the EU trusted us with the first and only Horizon 2020 project on biopesticides: BLOSTER. A project that proves our commitment to real sustainability. We replace synthetic molecules that are harmful to human health and the environment to obtain our fully natural biopesticides that are just as effective as synthetic chemicals and offer the maximum phytosanitary security. Economic impact, high quality and real sustainability, in contrast with conventional pesticides.
We develop soil probiotics capable of promoting plant development and production in row crops, reducing the use of fertilizers and increasing yield quality naturally. A profitable, safe and natural strategy.
SMART-O-LIVE
We are looking for olive trees that meet all our demands, zero residues, sustainability and healthiness, applying different project lines that cover the overcoming of stress periods, as well as pest and disease control. And for that, we use Artificial Intelligence through our LINNA® platform. This project includes Next Generation funding. Sustainable olive oils, which are healthier in the agroindustry of the future.
CARINA
The EU hast recently granted us our first Horizon Europe project for the development of a bioherbicide based on the oil of two different seeds (carinata and camelina). It is another step forward in our search for a natural product with the same efficacy and cost as glyphosate. We can also revaluate the proteins of these seeds for animal nutrition and bioplastic production.
SEANDER
The main objective of the SEANDER project is to identify and value new sources of raw materials of marine origin that are sustainable and not currently used, with application in the agri-food industry, as well as in the area of biopesticides.
CONDEMOR
Pesticide misuse has eliminated the life in our soils. An increasing loss of biodiversity, desertification and the severe modification or perturbation of microorganisms are some of the effects caused by those pesticides. Our CONDEMOOR project studies soil microorganisms, in an attempt to restore the soils’ natural balance. CONDEMOOR is our signature project in the probiotics line, which aims to increase crop yield and improve the fruits’ organoleptic properties. An alternative solution to the use of agrochemical inputs that are harmful to the environment, which also incorporates a biostimulant and biopesticidal effect. It is a win-win strategy: Higher competitiveness for the producer and a positive effect on the environment by reducing the incidence of phytopathogens and the use of chemical fertilizers.
WATER 2 RETURN
Phosphorus is one of the most important macronutrients in agricultural production, but did you know that it is likely to disappear? And there is no other nutrient to replace it. With WATER 2 RETURN, we aim to revaluate phosphorus, promoting its recovery and recirculation.
PHAGEFIRE
The European Union is now entrusting us with a bigger challenge: Find a solution to a problem that not even synthetic chemistry has been able to answer yet. PHAGEFIRE is our very own quest to find a fully natural biopesticidal solution for fire blight, a devastating disease affecting mainly pome fruit trees, which causes significant production and economic losses.
BIOPOLIM-A
Did you realize that tomatoes, bananas and avocados ripen too fast? They are called climacteric fruits that continue their ripening process by releasing ethylene after harvest. When they are packaged, this gas accelerates fruit ripening and reduces its post-harvest life. The project aimed to find a way to degrade that ethylene, so that the products last longer.
KA-OX
Ozone goes often unnoticed, but it is one of the biggest problems in open-field agriculture and causes significant losses in terms of productivity. Our KA-OX project resulted in different antioxidant solutions that eliminated this invisible enemy in fruit trees, open-field vegetable crops, and row crops, preventing the loss of productivity caused by ozone due to climate change.
MYCOCAP
We love mycorrizhae. For us, these fungi are at the very basis of agriculture. We have a deep understanding of how they work, and we have become the largest producer of mycorrhizae in the world. But we still have a lot to learn if we want to match the ideal fungal strains to specific crop types, with the aim to increase water and nutrient uptake, enhance the natural defenses of crops against nematodes and pests, obtain more vigorous and productive plants, etc. MYCOCAP allowed us to expand our strain collection and increase our knowledge in the field.
MIPLASCOE
We strongly believe that we can reduce our impact on the environment by developing our own bioplastics. We have managed to producer monomers through fermentation. These can be used to create biopolymers, wich in turn can be used to produce biodegradable packagings. We are now one step closer to reducing the use of conventional plastics in favor of bioplastics.
AEEF
This project aims to prolong the shelf life of fruits in the supermarket and the consumer’s fridge, especially of those ripening faster. We want to develop a unique packaging system for fruits capable of increasing their post-harvest life compared to other traditional packaging systems, through a novel system for capturing ethylene. Less waste and a higher economic impact guaranteed.
INGENIA
Can you imagine a healthier marmalade capable of offering an antioxidant activity? That is now possible thanks to our INGENIA project, in which we managed to find new uses for fruit waste, such as the pulp and the bones. Our research strategy consisted in extracting functional ingredients and antioxidants from those residues, which companies such as Helios later incorporate into their marmalades. The result: A healthier, mores sustainable product with antioxidant properties.
EOHUB
The Spanish Higher Council for Scientific Research (CSIC) invited us to participate in this European project due to ouf expertise in the use of essential oils for the development of biopesticides. By participating, we showed our support for this kind of entrepreneurial initiatives in the field of biotechnology with this type of oils, through a technical training and support program for young scientists, while promoting innovation and conservation in this field.
AGROHEALTH
Did you know that soil microorganisms in the areas where fruits and vegetables are cultivated can determine the chemopreventive action of our food? We wanted to prove that, by applying specific microorganisms to the soil, we could increase the concentration of natural active ingredients for that specific purpose in selected fruits and vegetables. We are leading the way back to the good old Mediterranean diet. The use of disinfectants and insecticides had eliminated many of the microorganisms associated with that “healing” effect of our fruits and vegetables. AGROHEALTH develops biostimulants and probiotics for tomato crops, grapevines and avocado trees, increasing the concentration of chemopreventive compounds against different lines of tumor cells.
10.S3
We are looking for the tastiest, healthiest, and most sustainable tomato ever, but keeping it free of residues and guaranteeing its productivity and organoleptic properties. We worked hand in hand with global leaders like CASI, the largest tomato producer in Europe, in the identification of different tomato varieties that could meet those demands. But they were not as productive as other classic varieties. By using specific microorganisms adapted to tomato crops and bioinsecticides developed by Kimitec, we managed to increase their productivity in CASI farms. We also applied Kimitec’s biopesticide to all greenhouse walls to increase crop protection.
CRESCERE
Can we go beyond the limits of agriculture and contribute to other sectors? Challenge accepted! In line with our original goal of “changing the way food is produced”, this project aims to demonstrate that some secondary crops offer a high concentration of natural proteins. Could we make a veggie burger with the same level of tastiness? We believe we can.
BIOCHAIN
China chose us to lead their transition from synthetic chemicals to a greener system to protect the environment and their consumers’ health. BIOCHAIN develops natural biostimulants, pest-specific biopesticides and prebiotics capable of protecting and feeding major crops in China. Starting by the characterization of chinese soils to determine the microorganisms they contain, we can look for the most appropriate probiotics. Natural solutions travel far and fast.
AGRARIA
Artificial Intelligence applied to the value chain of agricultural production 2050. AGRARIA is the only project for Spanish agriculture supported by Next Generations funds, and based on the application of our Artificial Intelligence platform LINNA® for the development of biopesticides, together with Sylentis. We will also design a highly technological pest detection system with drones. Soon after the pest appears, we will apply our biopesticides, to limit their use. The future starts today.
BLOSTER
¿Te imaginas poder reutilizar el bulbo de dónde sale la flor del azafrán? Lo hicimos e incorporamos como materia prima un residuo de una industria agroalimentaria. Este bulbo tiene high concentration con activity biopesticide. Es el primer proyecto de biopesticidas que nos concedieron y que nos enseñó el camino para la investigación del área de biopesticidas.
WATER2RETURN
Un proyecto de economía circular aplicado a la agricultura: una solución integrada para el tratamiento de aguas residuales de matadero, ricas en nitratos, para su recuperación y reciclarlos de manera segura para su aplicación como biofertilizantes de alto valor. Nuestra Inteligencia Natural nos permite ofrecerte soluciones que aportan valor y rentabilidad a tu negocio, ¿hablamos?
ATILA
Glyphosate, a massively used chemical product, must be replaced. The European Union gave the green light to our ATILA project, which aims to design and develop a next-generation bioherbicide based on by-products of orange juice production. To meet the challenge, we will be supported by our Artificial Intelligence platform LINNA®. We protect human health and the environment.
EOCENE
Together with the industry leader Grupo Consentino, Kimitec is trying to find a monomer that may act as a precursor of the resin used to produce Silestone®. The project EOCENE is based on a circular economy approach, aiming to obtain monomers for the production of that final resin through a polymerization process. All components of this composite meterial will be based on renewable and sustainable sources, through the development of sustainable technologies applied to the recycling and revaluation process of the waste produced.
BIOPRO
This project aims to research and develop new food ingredients based on agrifood by-products from different collaborators (Argal, Mahou, Matarromera, Pet Food Group, etc.), through bioconversion and biorefinery processes. We inoculate fungi into those residues, and through their activity they produce ingredients that are functional for the organism. Circular economy, sustainability and health applied to products for human and animal nutrition.
BIOTAGUT
Again, we are using by-products from the agrifood industry for the sake of people nutrition. Could we identify a healthy pattern in gut microbiota, so that we can adapt our eating habits and improve our health? Yes we could! Through our BIOTAGUT project, we work on the smart design of ingredients and products with a positive impact on metabolic health, such as obesity, diabetes, hypertension, etc. Because health starts with nutrition.
PLANT FERT
The perfect definition of a win-win strategy: Enjoy a good beer and use the excess liquid and yeast left after its production process. The PLANT FERT project aims to add new value to that waste, tranforming it into sustainable, agricultural biofertilizers for organic agriculture. We are experts in revaluation projects based on a win-win approach. The agroindustry saves money in waste management by selling its by-products… And we reduce our impact on the environment.
NATURDEV
This project aims to minimize the residues produced by the agrifood industry, increasing sustainability by incorporating processes and technologies capable of increasing its competitiveness. The purpose of that waste (mushrooms, in this case) is to obtain biostimulants, leveraging on the by-products to achieve a positive economic and environmental impact.
BIOPOLIM-A
Fue el primer proyecto Interconecta en el que participamos. Puso las bases para nuestra experiencia en la obtención de monómeros, a partir del suero de leche. Con el objetivo de hacer biofilm.
MIPLASCOE
¿De la cáscara y pulpa se puede obtener un bioplástico? Sure they can! Es un proyecto de revalorización total que lidera García Carrión. Partimos del residuo de la producción del zumo de naranja. Este, se usa como medio de cultivo gracias a unas bacterias programadas para obtener monómeros. Una vez que lo hemos purificado, la empresa que fabrica los envases crea el polímero usado en la producción de estos envases para zumos.
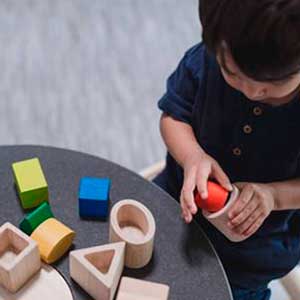
Research Areas
SAR
How can the child know in advance the shape that fits every mold? What if we changed the shape of the different pieces?
This is an exagerated simplification of the existing relationship between Chemistry and Biology.
The paradigm of how the smallest change in chemical structure may have a decisive impact on bioactivity is represented by the steroid hormones estrone (E1), estradiol (E2) and estriol (E3). The codes above refer to the number of hydroxyl groups (-OH) in each molecule.
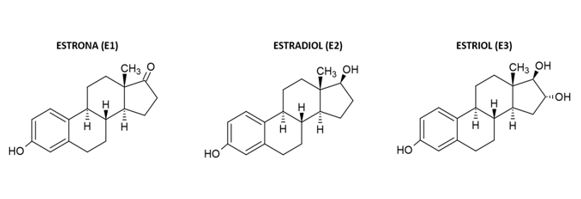
As shown in the picture, structural differences are minimal, but the biological impact is massive: Estradiol (E2) is 10 times stronger than estrone (E1) and 100 times stronger than estriol (E3).
Ref: Labhart, A. Clinical Endocrinology: Theory and Practice; Springer Science & Business Media, 2012; ISBN 978-3-642-96158-8.
Qualitative research on the chemical structure of molecules and their relationship with the physical and bioactive properties they possess is known as SAR (Structure-Activity Relationship). Crum-Brown and Fraser were the first researchers that described, in 1868, how the paralyzing capacity of a group of secondary metabolites of plants (the alkaloids strychnine, morphine, codeine and atropine, to be precise) was qualitatively related to the molecular structure of these compounds.
Ref: Brown AC, Fraser TR. On the Connection between Chemical Constitution and Physiological Action; with special reference to the Physiological Action of the Salts of the Ammonium Bases derived from Strychnia, Brucia, Thebaia, Codeia, Morphia, and Nicotia. J Anat Physiol.
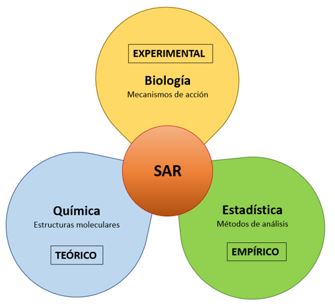
The relationship between the physicochemical properties and biological activity of a molecule can also be expressed through a mathematical expression. This allows to obtain quantitative data on the contribution of each functional group forming the compound to its bioactivity or reactivity, allowing to predict properties and activities after the in silico structural modification of the compound. These developments and processes are known as QSAR (Quantitative Structure-Activity Relationship) and its most general mathematical expression would be:
Activity = f (physicochemical properties and/or structural properties)
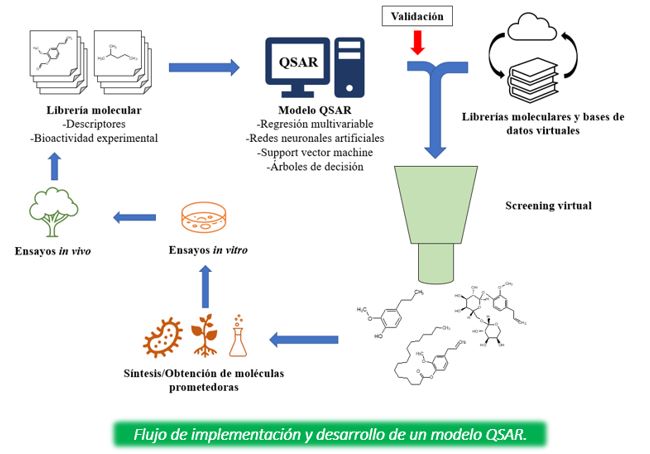
In synthetic chemistry, these approaches aim at increasing or reducing the affinity of the designed compound for the active or binding site to a ligand of specific, identified and structurally described proteins. Usually, these molecular designs aim to enhance the pharmacological effects*, such as the anti-carcinogenic action, or to reduce the toxicity of the molecules of interest.
Regarding applied natural chemistry, the study of the SAR/QSAR is more complex, since most receptors or targets involved in the interaction with natural molecules or the synergistic effects produced by mixing them are unknown. These models offer promising results, underlining the huge potential of this multidisciplinary branch as a powerful tool for the development of complex natural products that are more effective, economically profitable and harmless to both the environment and human health.
Considering the challenges and the described scenario, both SAR/QSAR methodologies aim to eliminate the random components in the design or discovery of new natural products showing a similar or even a greater biological activity compared to synthetic chemicals.
By establishing a mathematical relationship in the form of an equation, without necessarily knowing the structure of the molecular target, it is possible to deduce the bioactivity of new natural compounds without testing, drastically shortening the transition from synthetic chemistry to natural chemistry.
Research Areas
By-Product Revaluation
As a result of the continuous development and growth of the world’s population over the last century, the has been an increase of all kind of waste, especially in industries such as agriculture, aquaculture, livestock farming, the food industry and cosmetics. Before, this waste was burnt or left to degrade in the fields. Currently, environmental concerns and the need to look for renewable materials for the development of different products are pointing towards plant residues as a new source of raw materials. This work, developed in our MAAVi Innovation Center, helps to reduce operating expenses, offering a solution for the management of the waste derived from the company’s activity.
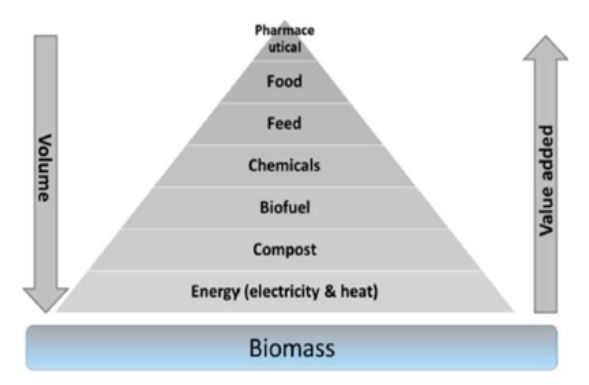
1st Generation
They are dumped into the rivers and oceans, or burnt.
2nd Generation
They are sent to a treatment plant, which increases operating costs for the company and still implies a significant impact on the environment.
3rd Generation
Using an R&D approach, waste is given a second life before it is sent to the treatment plant. Thus, the operating account of the company is not affected and the environmental impact of industrial activities is minimized.
“Turning a problem into an opportunity”
Social awareness has been followed by a series of official policies aimed at reducing, reusing and recycling said by-products, creating a niche market for companies that are capable of leveraging on the value provided by that waste.
The use of this waste may be quite straightforward, with not much added value, such as: Compost for the development of fertilizers or production of biofuels, biomethane, etc. But also highly technological, with a higher added value: Extraction of active biomolecules for the production of nutraceuticals; pectins for the food industry; production of nanocellulose for the cosmetics industry, textile applications, adhesives, etc.; extraction of hemicellulose for drug encapsulation, among others.
“MAAVi Lab Model”
Here at MAAVi we work hand in hand with large agricultural producers and the agroindustry. We evaluate their waste, both in terms of quantity and quality: Type of waste, annual volume, seasonality, origin of that waste, chemical composition, biological stability, potential proven applications, etc.
For applications in the field of agriculture, we organize agronomic trials; however, for other applications unrelated to agriculture, we involve third companies with a potential interest in the products.
All of it after studying the technical and economical viability of the project.
Research Areas
Phytochemistry
Plants are the largest biofactory of molecules known to date. According to estimates, over 400,000 plant species have been identified so far, which accounts for only 3% of all plant biodiversity. Any of those species is capable of producing from 5,000 up to tens of thousands of different compounds by itself. If we consider all the possible combinations between those compounds, we are literally speaking of a nearly infinite chemical space (thousands of millions of molecules). This chemical diversity is the key to plant survival throughout millions of years of evolution, and it is considered the largest source of active substances to which all kinds of biological properties are attributed.
In our Botany Department, we study and decode the chemical diversity of plants, to understand the interaction between different molecules. Thus, we can design new combinations targeting a broad spectrum of biological activities against numerous challenges.
The chemical space
The natural chemical space encompasses all the possible combinations of molecular structures found in nature. This is nearly infinite (thousands of millions of compounds), 95% unknown, and its largest known source of compounds are plants (over 70%). We develop a collaborative network with the largest centers dedicated to plant biodiversity in the world.
We can extract molecules by applying an innovative amplification node (hipervínculo a apartado Nodo de amplificación de otra sección) to the plant matrix, which consists of a unique combination of technological and biotechnological sequential processes. We proceed to their identification and structural elucidation through combined chromatographic and spectrographic techniques (hipervínculo a apartado Analítica) and chemometric analysis.
We define and isolate the marker compounds of the biological activity through advanced techniques based on bioassay-guided chemical fractionation, and analyze the results using chemometrics and artificial intelligence (hipervínculo apartado IA diferente al anterior)
We boost the power of nature through in vitro culture techniques, genetic enhancement and selective breeding of wild chemotypes.
We increase the diversity and functionality by selecting the leading natural molecules, using SAR studies and artificial intelligence algorithms.
We optimize the biological and toxicological properties of the molecules resulting from the targeted design of multifunctional synergies.
The chemical principles supporting our product and the responsible use of natural resources are important to us.
Natural chemistry principles supporting the product
Our chemical processes are based on molecules that are, or at least may be found in nature.
We use:
- Molecules that may be obtained through direct isolation of their natural source or through a biological process,
- Molecules that are equivalent to their natural counterpart (nature identical), and obtained through biobased processes from natural raw materials or processes where this technology is applied.
We do not use:
- Synthetic molecules that are not found in nature,
- Molecules wit a high risk of persistence, bioaccumulation and/or toxicity (POC, PBT).
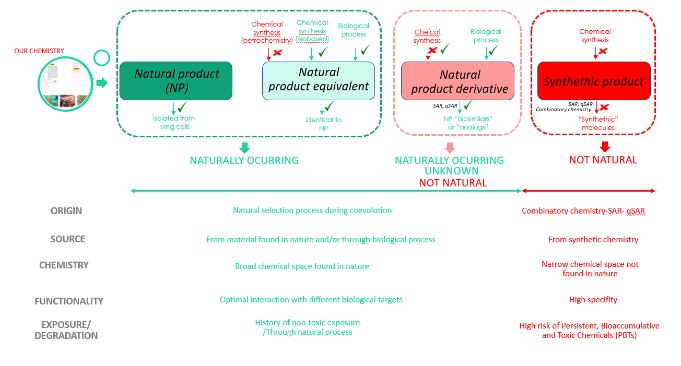
Responsible Use
We use natural resources responsibly. And we do it on three different levels
Raw material
- We comply with the Nagoya Protocol and the dispositions of the Convention on Biological Diversity
- We do not work with GMO
- We perform quality control both on the source (biodiversity centers) and on the target
Manufacturing Process
- We use biological and biotechnological processes
- We identify chemical and biological markers
- We identify and quantify any impurities
- Homogeneity verified through 5-batch analyses
- Validated methods for analysis and quality control
Molecular structure
- Proprietary methods for demonstration of occurrence in nature
- Predictive analysis to identify risks to humans and other non-targets
How do we understand multifunctional synergies
A synergistic effect consists in finding a molecular combination that offers a superior biological effect compared to the one achieved by the simple addition of the different components. This is a solid approach applied in numerous areas of the Medicine and Pharmacology domains. We are applying the same approach to other areas, such as Agriculture, Aquaculture, Livestock Farming, the Food Industry and Cosmetics.
We look for synergies to: Increase efficacy, lower application rates and active ingredient exposure levels, expand the “multitarget” spectrum of action, amplify action mechanisms, improve physicochemical properties, promote systemic activity and residuality, and meet any other requirements.
We are experts in the design of synergistic combinations of natural molecules. We are different in that (1) we study natural synergies and (2) we design exclusive combinations using an interdisciplinary approach comprising Botany, Microbiology, Chemistry, Biology, Bioinformatics, and Mathematics.
We begin by determining all of the chemical space contained within the plant. We use guided bioassays to perform chemical fractionation, in which each chemically characterized fraction is subjected to different biological challenges. In this manner, we can determine the function (synergistic, additive, cooperative, and/or antagonistic) of each chemical entity with regard to the crude extract’s activity levels.
The key to our success is that we understand how to extrapolate natural synergies into new combinatorial matrices. We use the Gibbs Triangle to construct a matrix with over 100 secondary and tertiary interactions between the chemical entities of the chosen marker molecules based on sublethal concentrations (less than LD50 or EC50). Using a phenotyping analysis, the efficacy levels of each combination are compared to those of each individual molecule, taking into consideration the respective 50% and 90% efficacy rates. The synergistic effects are validated on three levels: isobolographic analysis, the Limpel equation, and the Chou and Talalay combinatory index.
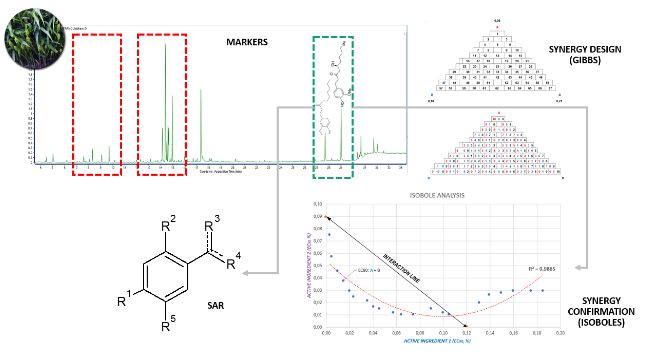
Research Areas
Plant Physiology
Plants are just as important as any other living organism on the planet. Plants are essentially the reason that most organisms survive on Earth. They provide us with the oxygen we breathe and the food we eat.
Plant Physiology is a field of Science that studies the structure and function of plants and their components.
Plant physiology is the study of all aspects of plant biology at the structural, molecular, and systemic levels, as well as their interactions with the environment (ecophysiology).
It combines information from a variety of scientific disciplines, including physics, chemistry, biochemistry, genetics, and molecular biology, to better understand how plants develop and survive within different environments.
It relies on a number of techniques and tools to assess a plant’s health and performance under a variety of cultivation or development conditions.
In agriculture, it is essential to understand how the plants of a specific crop function in order to analyze, recover, and enhance their performance and yield.
Throughout their life cycle, plants are exposed to many kinds of stressors that jeopardize their ability to survive and, in the case of crops, result in yield losses. These stressors can be biotic (a pest or disease) or abiotic (a drought, a frost, for example).
We can more accurately design products targeted at avoiding, overcoming, and recovering from these stressors if we understand plant physiology. It is a component of what we do at Maavi.
How can we determine if a plant is stressed and what changes take place?
Environmental stressors such as high temperatures, frost, drought, changes in light intensity, salinity, nutritional deficiencies, heavy metals, herbicides, ozone, and so on affect plant function directly or indirectly, influencing fluorescence emission. Multiple techniques can be used to reveal response mechanisms, evaluate stress responses, and pinpoint specific pollutants and their source.
Some of these techniques are:
-
Chlorophyll fluorescence
-
IRGA: infrared gas analyzer.
-
Biochemical techniques: MDA, quantification
Research Areas
Microalgal biotechnology
Microalgae are unicellular plants that we turn into tiny natural factories, from which we extract millions of compounds with high biotechnological value.
Unicellular plants
Microalgae belong to the plant kingdom and, like higher plants, they can be autotrophic, cosmopolitan organisms that accumulate similar secondary metabolites in response to specific environmental conditions. But what characteristics have made them appealing in the area of biotechnology?
- Not being obligate autotrophs, because these microbes, in addition to photosynthesis, can use organic carbon sources, resulting in heterotrophic nutrition, or combine both methods to obtain energy, resulting in mixotrophic nutrition.
- They can tolerate extremely harsh conditions in terms of pH, salinity, and/or temperature due to certain physiological characteristics that enable them to thrive in extreme environments such as glaciers, deserts, or even unusual locations such as burial sites.
Given the aforementioned, it is possible to direct the accumulation of secondary metabolites in microalgae with specific physiological properties, turning them into tiny biotechnological factories.
Secondary metabolites
Microalgae have different physiological reactions to different exogenous conditions.
The phenotypic behavior of microalgae in response to external factors changes their phytohormone, pigment, and fatty acid content, as well as their biopesticide activity.
At MAAVi, we study how organisms behave in their natural environments by identifying and replicating under controlled settings the conditions that result in the activation of metabolic pathways for compounds of biotechnological interest.
Natural factories
At MAAVi, we value natural diversity; as a result, we prospect, characterize, and optimize the accumulation of bioactive compounds in order to find the best production system on a larger scale that balances the amount of biomass generated and the accumulation of metabolites of interest, at the lowest possible production cost.
- Identifying bioactives in microalgae species
We take into account the wide variety of microalgae that exist (over 30,000 species), which is why we favor strains of microalgae that have been largely unexplored, bearing in mind that they are not all the same and taking advantage of their phenotypic plasticity.
- High resolution characterization
We can extract molecules by applying an innovative amplification node to the plant matrix, which consists of a unique combination of technological and biotechnological sequential processes. We proceed to their identification and structural elucidation through combined chromatographic and spectrographic techniques and chemometric analysis.
We increase the diversity and functionality by selecting the leading natural molecules, using SAR studies and artificial intelligence algorithms.
We optimize the biological and toxicological properties of the molecules resulting from the targeted design of multifunctional synergies.
- Optimization with non-invasive strategies
We seek to add value to what is natural in a sustainable manner. A strain must be able to maintain an equilibrium between its ability to generate biomass and accumulate metabolites of interest, while remaining scalable and profitable in terms of production processes. To do this, we focus on adaptive evolution strategies in the laboratory.
- Testing and scalability systems
We have several 2L controlled culture systems (mixing, aeration, temperature, pH, and lighting), which enable us to produce enough biomass for later analysis, as well as 20L reactors that allow us to begin the production of cultures with which to inoculate our semi-industrial unit.
The semi-industrial unit, on the other hand, includes different processes that allow us to increase the quantity of biomass in the cultures. These include open culture (775 L raceway) and closed culture, with tubular (700L), cylindrical and flat (500 L) photobioreactors, and 4 cylindrical systems (150 L each). Each PBR has sensors that monitor the pH and temperature of the culture as well as other control and monitoring parameters. This information can be electronically analyzed through a computer program, allowing for remote access to the data in real time for quick decision-making and regulation of growth conditions.
It should be noted that our pilot plant consists of a system of independent feeding and harvesting pumps, which allow operation at different flow rates or operating regimes (continuous, semi-continuous or batch). Therefore, the structure of this design allows us to compare each system and look for the one that is most appropriate for each strain and target metabolite.
Biotechnological value
Las microalgas, junto a las demás fuentes naturales con las que trabajamos en Maavi (4HEALTH) representan un patrimonio biológico importante. Desde ellas obtenemos moléculas con las que desarrollamos nuevos e innovadores productos para los distintos mercados a los que ayudamos en su transición hacia lo natural.
Y es que las microalgas presentan un sinfín de posibilidades y en Maavi estamos alertas a las nuevas tendencias investigativas para abordarlos y ensayarlos. Y es que uno de nuestros aspectos que nos diferencian de cualquier otro centro de investigación microalgal es que contamos con gran cantidad de plataformas de ensayo, donde realizamos desde ensayos in vitro hasta pruebas en campo. Además, nos apoyamos en nuestras redes de colaboración nacionales e internacionales, académicas, investigativas y comerciales, logrando así un escenario multidisciplinario a partir del cual generar nuestros desarrollos biotecnológicos.
Microalgae, together with the other natural sources with which we work at MAAVi (4HEALTH)constitute a significant biological legacy. They provide us with the molecules we need to create new, cutting-edge products for various markets as we support their transition toward natural alternatives.
This is due to the fact that microalgae offer infinite possibilities and at MAAVi, we are continuously on the lookout for new research trends to address and test. We also have a number of testing platforms where we can carry out everything from in vitro tests to field trials, which distinguishes us from other microalgal research facilities. Additionally, we rely on our academic, research, and business national and foreign cooperation networks to create a multidisciplinary framework from which to develop our biotechnological innovations.
Products
Among the products developed by MAAVi using microalgae, Seawex stands out as the only all-natural product based on the joint biostimulant action of seaweed and microalgae. It is an active source of natural phytohormones, such as cytokinins, auxins and gibberellins, but also of other components, such as free amino acids, vitamins, carbohydrates and antioxidants, which promote stress resistance and prepare the crop for periods of high energy demand or phenological stage changes. Its perfect combination optimizes all metabolic and physiological processes, maximizing crop yield.
Research Areas
Priming
Plants have developed their own defense mechanisms, similar to how humans have developed an immune system against pathogens.
Do you agree that it is exciting to try to understand how an organism can spend its entire life in one location without having the choice of moving elsewhere to escape adverse weather conditions or pathogens?
Can we argue that plants have an immune system? Do plants have an immunological memory capable of recalling attacks that they have previously overcome?
The Evolution of Plant Defense Systems
Priming is an evolution of a plant’s defense mechanism.
According to Martínez-Medina and collaborators (2016), it is the physiological state in which plants are prepared or pre-conditioned for a more efficient activation of their defenses against environmental challenges, both biotic and abiotic.
How do plants train or develop their defense mechanisms?
In the natural world, when a plant senses a threat, it activates its defense mechanisms in response to the threat it detected. These stimuli can include a pathogen infecting plant cells, a beneficial organism interacting with the plant, mechanical friction with a human or animal, and so on.
MAAVi conducts research to prepare plants in advance, before they detect a threat or attack, using natural molecules known as “elicitors”. We are talking about “elicited” or “primed” plants.
These elicitors will boost plant cell alertness, enabling them to trigger defense mechanisms more effectively when the real threat emerges.
Would you like to know which kind of elicitors we research at MAAVi?
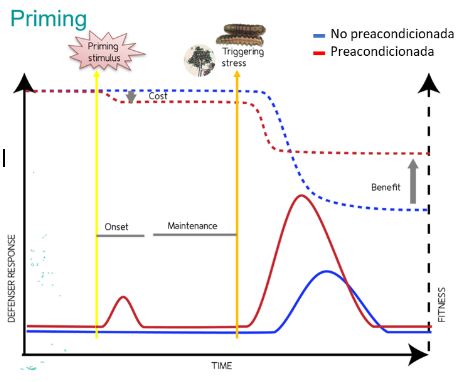
PRIMING elicitors allow us to rely on plants’ inherent responses by:
- Being a component of a more complex composition to improve efficacy
- Reducing the use of phytosanitary products by alternating applications
Since the different pathways that make up plant immunity are linked and react to the multiple stresses that these plants experience at the same time (a plant attacked by a pathogen may be suffering from salinity or temperature stress at the same time), PRIMING activation is metabolically more effective than the direct activation of isolated pathways.
Advantages of PRIMING
– For each stressor or challenge that plants face, the main biologically preset pathways are activated.
– These pathways are only activated when the plant experiences the specified stress, not before.
– The activation of plant natural defenses is more intense and maintained over time.
– It implies that the plants’ energy balance is improved because the metabolic cost of activating defense systems happens only when necessary.
Can we argue that plants have an immune system or immunological memory?
Not strictly speaking. Although various sources refer to PRIMING as a plant’s immune system, the truth is that the signals that travel through the xylem/phloem vanish quickly after serving their purpose, whereas a human is protected for a longer period after receiving a vaccine.
Plants, like Dory, have a short term memory and react to challenges by expending energy to defend themselves; once the challenge is passed, the plant returns to its resting state. Even when facing the same challenge, the defense mechanism must be activated from scratch.
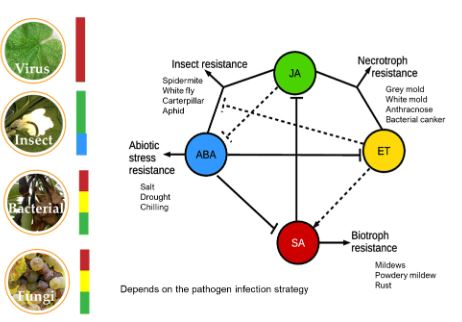
Plants can recognize certain molecular patterns and respond accordingly. These substances can be categorized as MAMPs, PAMPs, or DAMPs depending on whether they are derived from microbes, pathogens, or plant cell damage.
Plants, in addition to these molecules, can react to certain chemical or environmental stimuli.
Some natural compounds, such as oligosaccharides, glycosides, amides, vitamins, carboxylic acids, and aromatic compounds, have already been linked to the Priming phenomenon as growth boosters, activators, or inducers of plant defenses.
In general, plants handle natural compounds better than most synthetic compounds analyzed. (Iriti et al., 2010; Noutoshi et al., 2012; Aranega-Bou et al., 2014).
As a result, at MAAVi Innovation Center, we look for these compounds within the primary natural sources that our solutions are based on:
– from Botany, for example: Milsana (extracted from Reynoutria sachalinensis), oligogalacturonides, burdock fructooligosaccharides…
– from Microalgae, carrageenans, fucans, ulvans, laminarin…
– from Microbiology, flagellin, harpin-proteins, lipopeptides, β-glucans, chitosan, chitin, ergosterol…
Would you like to know how we uncover novel Priming elicitors?
The general scheme of the experimental design entails treating healthy plants with different ingredients or formulations to identify enzymatic activities that indicate the condition of defense mechanisms.
Samples are collected for evaluation of defensive mechanism performance at least 24 hours after the treatments —to see if they revert to their basal state after priming stimulation— and a few hours after infection. This period varies depending on the pathosystem and enzyme in question, and an effort is made to determine the point of maximum active defense expression.
The infection is sustained until the symptoms are measurable, with variations showing between primed plants and control plants.
Different enzymatic activities are processed and evaluated from tissue samples of various kinds. They may be:
– Phenylalanine ammonium lyase (PAL), a salicylic (SA) pathway marker
– Leucine amino peptidase (LAP), a jasmonate (JA) pathway marker
– Reactive Oxygen Species (ROS) and Nitrogen Species (RNS)
– Peroxidases (PX)
– Superoxide dismutase (SOD)
– Chitinases (CHI)
– β-1,3- Glucanases
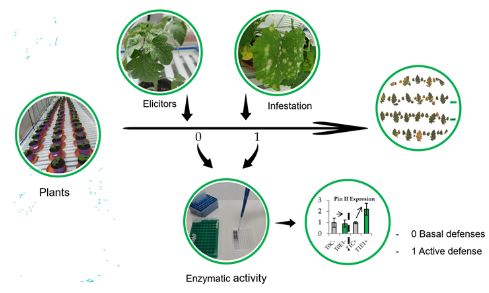
Research Areas
Enthomology
Insect Breeding and Targeted Insect Testing Area.
MAAVi has pest insect breeding systems in place that allow us to undertake biopesticide trials throughout the year.
Discover where we do research with our “allies” to develop our solutions..
Enthomology is the scientific study of insects, which account for two-thirds of all documented species worldwide (1.3 million in total).
This area of MAAVi is in charge of breeding the insect pests that are the target of our biocontrol solutions.
Once we provide optimal conditions, insect populations become established in our breeding boxes, with some species reaching densities of up to 10,000 individuals.
Then, as part of our Hit 2 Lead process, we subject them to several testing phases.
Candidate Primary Trials:
-
Contact mortality on the pest’s stage of development that is most aggressive for the crop.
-
We set efficacy standards of 75% or above for candidates to advance to the next round of trials.
-
Trials performed in vitro on plant material.
Secondary trials of Hits, Leads, Synergies and Preformulates or pest behavior trials:
-
Settlement inhibition
-
Oviposition inhibition
-
Feeding inhibition
-
Performend “in planta”
-
Dose–response assessment
Therefore, we conduct studies at all phases of pest life cycles in order to define modes of action and identify population-reduction mechanisms.
En MAAVI contamos con las siguientes instalaciones de cría y ensayos:
-
We have the following breeding and testing facilities at MAAVi:
-
200 m2 of greenhouses divided into:
- Ten 20m2 sub-units with overhead ventilation, air extractors, and temperature control.
- Two 20m2 growth chambers with temperature, humidity, and photoperiod control.
-
80 m2 of in vitro and in planta testing area.
We conduct over 600 trials per week
Research Areas
Phenotyping
The term phenotype refers to any observable characteristic of a plant, such as its height, number and size of leaves, level of chlorophyll, etc. This characteristic or attribute is the result of the interaction between the genotype of the plant and its environment throughout development. Plant phenotyping analyzes how a plant’s genetic make-up interacts with its environment during development to produce specific traits or characteristics. Our platform LINNA, enables precise mass phenotyping of plants through the use of non-invasive approaches based on digital images and sensors. Digital images obtained with various sensors (hyperlink to click 2) are processed at the pixel level and transformed into binary code by our artificial vision system, translating nature into data understandable by LINNA® prediction models. This allows us to evaluate, for example, the response of plants under different types of environmental stress, as well as the influence of biostimulants and bioherbicides on their development and growth. The evaluation of individual qualitative and quantitative attributes serves as a basis for assessing complex characteristics.
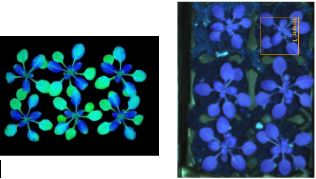
PAM Images. Image of non-photochemical quenching parameter NPQ of Arabidopsis plants..
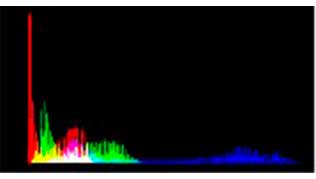
Hyperspectral image. On the right, histogram for the selected region.
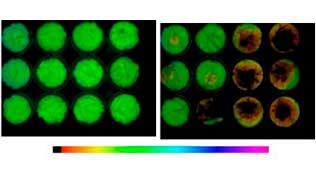
Tests on untreated leaf discs (left) and those treated with Phytophthora (right). Images corresponding to parameter Y(II).
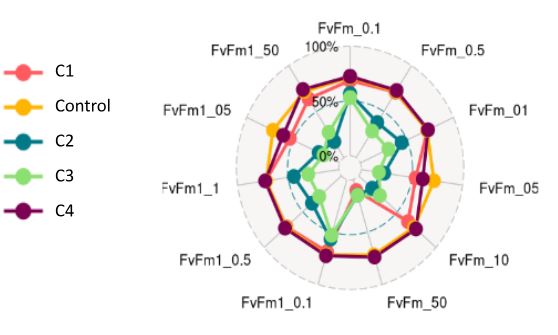
Effect caused by different bioherbicides on Arabidopsis plants. Diagrams based on chlorophyll fluorescence images displaying the values corresponding to PSII’s best performance in the dark at various bioherbicide concentrations (FvFm).
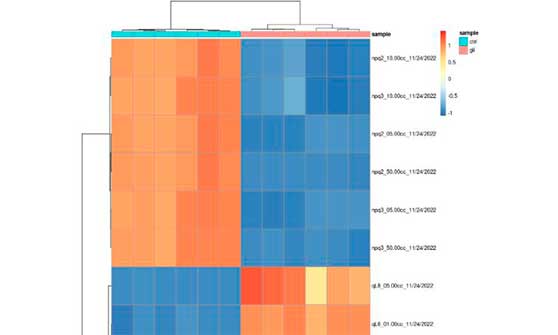
Hierarchical cluster generated from chlorophyll fluorescence images. The most important photosynthetic response metrics in plants treated with a herbicide (glil) or not treated (ctrl) are shown.
We have a phenotyping platform at LINNA which offers automated measurements using multiple sensors. This high-performance integrative phenotyping integrates several automated, simultaneous, and non-destructive plant evaluation approaches. This provides information about the growth, morphology and physiology of plants. The high performance allows us to analyze plants on a broad scale and use statistics to identify subtle yet significant variations between different treatments or genotypes.
Images obtained with visible red-green-blue (RGB) sensors are used to measure visual parameters in the LINNA platform; hyperspectral sensors are used to obtain information on the internal structure of the plant and its content of compounds of interest (pigments, proteins, carbohydrates, water, nitrogen, lignin, and so on); and chlorophyll fluorescence sensors are used to determine photosynthetic efficiency. Given that phenotyping entails a cascade of events affecting gene expression (transcriptome), data from the LINNA transcriptomics platform are integrated into the phenotyping process. Through data analytics and artificial intelligence-based algorithms , LINNA is able to collect key data for the development of natural solutions at MAAVi.
This data integration enables us to predict or identify the variables causing variations in crop physiology and morphology. For instance, it helps in the identification of a herbicide’s mode of action, changes that lead to an improvement in crop yield, and the subsequent development of new products.
Simultaneously, the system reduces research time, increases accumulated knowledge, and, most importantly, reduces failure by making a faster and more accurate transition to natural molecules and compounds to replace pesticides with biopesticides, artificial preservatives with natural ones, or find new protein sources for food.
Would you want to learn more about MAAVi’s phenotyping platform?
RGB Images
It is used to quickly and accurately assess growth rate, leaf area, projected area, leaf greenness, leaf damage, senescence, among others. It is also possible to create growth curves using models and analytics.
Chlorophyll fluorescence images.
PAM fluorometry and high-sensitivity CCD cameras used for chlorophyll fluorescence imaging provide quantitative information on the quantum yield of photosynthetic energy conversion. This technique also allows for the separation of photochemical and non-photochemical events in leaves. This sensor offers 17 essential chlorophyll fluorescence metrics in addition to chlorophyll fluorescence imaging. This method may be used to collect images of photosynthetic activity and associated spatiotemporal oscillations. The fluorescence parameter Ft is continuously monitored. Fo and Fm are evaluated following adaptation to darkness. Using the saturation pulse approach, various characteristics relating to fluorescence quenching are derived from these. Fv / Fm (maximum quantum yield of PS II following darkness acclimation), Y(II) (quantum yield of PS II during illumination), regulated energy dissipation quantum yields Y(NPQ) and unregulated Y(NO), apparent electron transport rate (ETR), non-photochemical quenching (NPQ), and photochemical quenching (qP) images are obtained.
Hyperspectral images.
We have two cameras. The Specim FX10 operates in a line scan mode in the 400-1000 nm visible and near infrared (VNIR) range, and color optimized in the 400-780 nm region. Specim FX17 gathers hyperspectral data in the near infrared NIR range (900 to 1700 nm) while operating in line scan mode. Our analytical program allows us to calculate around 150 metrics such as NDVI, GDVI, NDLI, WI, NDWI, Crt, and LSI.
We may examine biochemical properties in plants using hyperspectral technologies, such as measuring water content, changes in pigment composition, moisture content, proteins, carbohydrates, sugars, and many more. We can also determine the extent of damage caused by insects and bacteria.
Research Areas
Formulation and systemicity
We combine ingredients and insights to create novel solutions from natural molecules and microorganisms.
We combine molecules with complex mixtures of multiple chemical identities derived from natural sources. We also design solutions that contain helpful microorganisms including bacteria, fungi, microalgae, and viruses. In this area, we develop exclusive formulas that are stable, compatible, persistent, and with improved efficacy for application in Agriculture, Livestock, Food Industry, Aquaculture, and Cosmetics.
Formulation technology
We combine ingredients and insights to create novel solutions from natural molecules and microorganisms.
We combine molecules with complex mixtures of multiple chemical identities derived from natural sources. We also design solutions that contain helpful microorganisms including bacteria, fungi, microalgae, and viruses. In this area, we develop exclusive formulas that are stable, compatible, persistent, and with improved efficacy for application in Agriculture, Livestock, Food Industry, Aquaculture, and Cosmetics.
Natural product formulation has the significant problem of integrating a broad and complex chemical diversity with substantial variances in their physicochemical qualities. It is an important step in the product development process that may make-or-break a project.
We begin the formulation development process with an in-depth understanding of the chemistry underlying each extract, synergistic combination, specific molecule, or microorganism. Each project and product requires its own formulation and comprehensive analysis. As a result, formulations may be developed employing a variety of technologies, including drying methods (spray-drying, freeze-drying), emulsions, emulsifiable concentrates, and concentrated suspensions, among others.
The challenge in microbiological formulations is to keep cells viable at high concentrations while also preserving the production capacity of molecules of interest, because cells that are not properly formulated can be exposed to unfavorable conditions during product production, distribution, and storage.
Our formulation technology innovation focuses on the following areas:
- Research of new multifunctional adjuvants and/or co-formulants of natural origin.
- Development of encapsulation and/or protective technologies for microorganisms and sensitive molecules.
- Development of controlled release matrices.
- Development of natural molecules that act as selective carriers of active substances and which allow: (1) Penetrability, (2) Translaminarity, (3) Translocation and (4) Systemy.
Our formulations are developed with the desired characteristics of a product in mind. For instance, we can offer multiple strategies/solutions such as adhesive capacity, rain resistance, UV light protection, and phagostimulants, among others.
Our main objective is to maximize efficacy while also ensuring formulation stability. Among our processes, we implement:
- Stability tests
- Accelerated stability tests (CIPAC MT 46.4). Their objective to determine if the shelf life of the formulations is met under normal storage conditions.
- Real-time stability tests (storage stability-shelf life). Performed under predetermined storage conditions, for the duration of the product’s lifespan and in its original packaging.
- Low temperature stability tests. The objective is to determine how storage at low temperatures affects the formulation.
- Packaging compatibility tests. To verify that no unfavorable interactions occur between the formulation and its primary container.
- Homogeneity tests (5-batch analysis)
- Chemical compatibility tests with other products
Assessment testing of the formulations’ physicochemical behavior (solubility, dispersion, suspensibility, wettability, etc.).
- Effect residuality tests.
All of this allows us to guarantee that the products’ active and inert components satisfy technical standards and may be registered in line with the sector’s and current legislation.
Research Areas
Analytical Chemistry
We look for molecules, not extracts
Natural extracts are comprised of a huge number of molecules, the concentration of which can greatly vary depending on the type of plant, age, season of harvest, climate, etc. In this area, we monitor the active molecules to guarantee that the efficacy of our products is homogeneous.
The technology implemented in the area enables us to analyze the complete chemical composition of a natural extract and identify the molecules that are responsible for its activity. Additionally, we monitor the concentration of active molecules, thus allowing us to ensure the efficacy and, most importantly, the homogeneity of our products.
We adhere to a pharmaceutical manufacturing process that guarantees molecular homogeneity.
Natural products formerly provided non-homogenous activity; however, controlling this at the molecular level allows us to compete directly with synthetic chemicals.
We employ the most advanced mass spectrometry equipment together with chromatographic techniques (GC and LC) for carrying out the molecular analysis of natural extracts.
Using chromatography, we isolate every substance detected by mass spectrometers (single Quad and Q-TOF). The advantage of using this type of equipment is that it allows us to identify molecules without the need for analytical standards, and enables us to discover hundreds of different molecules in a single natural extract. Additionally, they allow us to explore the uncharted territory since, by combining the analytical findings with chemoinformatics techniques, we may discover novel molecules and correlate them to the activity of the extract.
Research Areas
Toxicology
At MAAVi Innovation Center, we are particularly mindful of the safety of the products that we manufacture. Toxicity is a dose-dependent phenomenon even in nature. Isn’t it fascinating? Keep reading
As a result, studying the toxicity of the products that we develop is a critical step in the manufacturing process. We employ cell cultures to determine the toxicity of the compounds we work with, as well as the levels at which they may be toxic.
MAAVi has a laboratory devoted to toxicological testing at the cellular level. The trials are conducted using a liver cell line, namely HepG2, cultivated in multiwell plates. This cell line was chosen since the liver is the first organ to be affected by the toxicity of any foreign substance to the body, and it has the greatest detoxifying ability. These tests are performed by evaluating a number of escalating dosages of the compound and using the XTT technique to measure the cytotoxicity the compound causes in the cells that receive each dose. The algorithms suggested by the Interagency Coordinating Committee on the Validation of Alternative Methods (ICCVAM) Acute Toxicity Working Group (ATWG) are then used to create a correlation between the components’ IC50x cytotoxicity and acute oral toxicity LD50 without the need of animal testing.
Research Areas
Scalability
The MAAVi Innovation Center is renowned for its scale-up infrastructure that enables the industrial replication of laboratory-tested processes. This potential for realistic scalability allows us to make much safer and more accurate decisions about a process’s exploitability, and takes us even closer to meeting the needs of the industry.
We have pilot plants with extraction tanks up to 3,000 liters, vacuum concentrators with 300 liters/hour of water and 800 liters/hour of ethanol concentration, fermenters that can hold 100 and 3,000 liters, centrifuges with a capacity of 250 liters/hour, membrane plants that can process up to 100 liters/hour (depending on pore size), and mixing and formulation tanks with a 500 liter capacity and high shear and emulsion systems.
Every process must be designed and tested in the lab, but if it cannot later be replicated at an industrial scale, it is absolutely useless. This is why we, at MAAVi, place such a high importance on our pilot plants. Based on the knowledge gained during the laboratory design of a process, we may execute it at the industrial level, providing us with vital information that will allow us to make investment decisions for the industrialization of a process. Parameters like temperature, viscosity, calorimetric properties, shear rate, gas exchange capacity, or the design of the facilities that will allow the laboratory-developed products to be manufactured, considering the geometry of the deposits, the heat exchange surfaces, the torque of the agitation motors, or the design of the agitators allow us to determine whether a process is technically feasible on an industrial scale, estimate the volume of investment that said process may require, how much energy it will consume, what volume and type of waste will be generated, and what automation capacity could be implemented. In addition to determining the workforce and its characteristics related to the process, what services will be required (steam, compressed air, gases, sterility level, hot water, chiller water, osmosis water, etc.), the required space or footprint for its implementation, the carbon footprint generated, and so on. In short, any information and experience that is as near to reality as possible can aid in estimating the ROI of a new process, as well as other environmental factors that are currently having a major impact.
Research Areas
Pyrolysis
Human observations of natural phenomena, such as wildfires, have led to the identification of mechanisms involved in the synthesis of molecules with high value in various sectors, such as molecules with biopesticide activity, biostimulant activity, or other qualities. These processes can be integrated under the umbrella of pyrolysis. For more information.
Our pyrolysis technique causes the thermal breakdown of a natural matrix under partial absence of oxygen, so that the components present are degraded by heat rather than combustion processes. The process is carried out in a system designed by Kimitec, which yields two types of products: on the one hand, a solid residue that remains after the procedure, known as biochar, and which has interesting characteristics that contribute to healthier soils. The volatile components created in the process, on the other hand, are collected, and it is in this fraction that molecules with strong activity are obtained at extremely low dosages.
This is done at relatively moderate temperatures (below 300 °C) in comparison to standard pyrolysis processes, which are conditions that most closely resemble natural fires. At these temperatures, the most common reactions are depolymerization, oxidation, dehydration, and decarboxylation. Additionally, the majority of the affected components are all those with low molecular weight, with amorphous cellulose and hemicellulose standing out among those with high molecular weight. Sound interesting? Keep reading.
Karrikinas (KAR), one of the most important by-products of pyrolysis processes, have the capacity to enhance seed germination in a variety of plant species. However, it has also been shown that KARs have an impact on a number of biological functions in addition to seed germination. Although KARs are produced by burning plant matter, it is not yet known if karrikinas are naturally produced by living organisms. Chemically speaking, the pyran ring of KAR is formed by the pyrolytic reaction of simple carbohydrates (xylose, glucose, or cellulose). Similar to this, the aminocarbonyl reactions of certain amino acids with glucose produce germination stimulators. On the other hand, germination is inhibited by the by-product of the reaction between glucose and cysteine. The combination with a higher concentration of glucose had a better germination activity, according to tests with varying ratios of amino acids and sugar.
Plants with KAR signaling system anomalies were shown to develop differently and exhibit a variety of morphological abnormalities. The discovery that KARs operate as growth regulators in plants has prompted scientists to look for an endogenous version of KARs, which they believe to be present in strigolactones. In any case, as demonstrated in the following scheme, both kinds of molecular structures are in charge of essential processes involved in plant growth.
Research Areas
Microbiology
Microorganisms and their entire metabolic diversity have evolved over the last 3.8-4.3 billion years, to perform very different functions in all terrestrial ecosystems.
In the area of microbiology, we study the genetic and metabolic potential of all these microorganisms in order to guide them to produce or perform the action of interest.
Fungi, bacteria, or viruses (such as bacteriophages) are used to produce compounds with plant biostimulation or biocontrol activity, as well as food additives, antibiotics, and microbial cells. As a consequence, the microorganisms we work with at MAAVi could be considered metabolic specialists, capable of flourishing in a wide range of substrates, synthesizing molecules of interest with high output, or biostimulating the plant when used as probiotics.
Microorganisms, prolific producers of bioactive metabolites (or molecules). We identify and optimize the metabolic capabilities of microorganisms. Our goal is to produce molecules of interest.
Fermentation
Learn more about the process
Keep reading
Probiótics
Learn more about the process
Keep reading
Phages
Learn more about the process
Keep reading
Symbionts
Learn more about the process
Keep reading
Fermentation
Fermentation is used to obtain microbial cells to develop probiotics or to produce specific molecules of interest. In this specific case, the fermentation process applies to any large-scale microbial process, whether or not it is fermentation in the biochemical sense. In fact, many of the fermentations we perform at MAAVi are aerobic. Fermentors vary in capacity from 1 to 5 liters to 3,000 liters. Fungi, bacteria, and bacteriophages are the most common microbes used in our fermentations. We perform both liquid and solid fermentations with various plant materials as a matrix.
Microorganisms must exhibit certain traits in order to be used in industrial-scale fermentations: 1) It must first be able to develop rapidly and synthesize the product in either a liquid or solid culture, and 2) it must be able to grow in a cost-effective culture medium. We use waste by-products from other industries in many of our fermentations, in collaboration with our revaluation vertical.
Our work in the area of fermentations
We integrate information from microbial genomics, culturomics, and bioinformatics to perform microbial fermentations with the goal of getting metabolites of interest.
To select a suitable microorganism for fermentation, an in silico search is carried out within the more than 10,000 genomes of bacteria that we have in the database. The microorganism with the ideal genetic potential for producing the desired molecule can be predicted using this search.
Once the microorganism has been defined in silico, we either acquire it from our own collection of more than XY microbial strains (which can generate different compounds with the potential for biocontrol or biostimulation) or from research institutions with which we frequently collaborate.
Following that, small-scale microfermentations are carried out to maximize the production of the desired metabolite. We can perform more than 1,000 microfermentations per week thanks to the system developed at MAAVi.
Chromatographs are used to assess these microfermentations by tracking the metabolite and its concentration in different settings. The bioreactors with the largest capacity are used once the production conditions for the metabolite have been determined. We can perform more than 5,000 fermentations annually in our 20 bioreactors, which range in capacity from 1 to 5 liters.
Probiotics
The importance of understanding and maintaining the microbiological homeostasis of plants. Is it a plant, an animal or a holobiont? In nature, plants are never alone, they coexist in close proximity to bacteria, fungi, viruses, and other microorganisms (what is known as the plant microbiota). Because of this, at MAAVi, we regard plants as members of holobionts, which is made up of a multicellular organism (the plant) and all of its ancillary microbes. We restore the microbiological equilibrium that plant systems have drastically deviated from as a consequence of extensive farming.
It is important to understand and maintain the plant’s microbiological homeostasis, in which the plant is in harmony not only with its surroundings but also with its microbiota, (what is known as eubiosis). Today, this equilibrium has been disrupted as a result of the unrestricted use of chemicals. This vertical’s objective is to restore plant systems to the microbiological equilibrium from which they have greatly deviated due to extensive farming. In order to achieve this, we choose, cultivate, and develop communities of probiotic microbes that biostimulate plant development, aid in mineral nutrition, and mitigate biotic and abiotic stress. We rely on resources like ecology, microbial genomics, and metagenomics to accomplish this.
Genomics and Artificial Intelligence. Our understanding of it at MAAVi.
Microbial genomics, in conjunction with artificial intelligence, allows us to understand the full metabolic potential of the microorganisms we have at MAAVi, as well as the full metabolic potential of microorganisms whose genomes are available in open access collections. This tool is very useful in three areas:
- When developing a probiotic or a microbial consortium with biopesticide activity, because it will identify microorganisms with a potential for the desired activity and will also create synergies in a consortium.
- To find microbes that produce bioactive molecules.
- It is also very helpful when setting up precision fermentations where the culture medium and fermentation parameters are pre-determined.
Many of the microbial inoculants available today yield highly inconsistent results, based on the plant and environment in which they are used. This is due to the microbial inoculant’s failure to colonize and establish itself in the growth medium in order to serve its purpose. The combination of metagenomics and artificial intelligence enables us to determine whether the applied microbial product was successful in establishing itself and changing the microbial community. Furthermore, by examining the microbial communities of more and less productive crops, we can find important species for microbial community structure and function, thereby improving plant productivity. This helps us in developing prebiotic products capable of promoting crop beneficial communities.
Use of metagenomics and microbial ecology to develop pre- and probiotics for crops and soil-directed products.
In nature, microorganisms never survive in isolation; rather, they exist in groups of microbial cells known as microbial communities that interact with one another, with other organisms, and with their surroundings. And we need to take that into consideration if we want to study them and understand their mechanisms. Additionally, the soil is the terrestrial ecosystem that presents the greatest biodiversity. In a typical edaphic bacterial population, there are between 107 and 109 cells per gram of soil, and anywhere from 20,000 and 40,000 distinct bacterial species may be present. These microbial communities play an important role in the carbon and nitrogen biogeochemical cycles, in the preservation of natural ecosystems, and in agricultural production. As a result, studying these edaphic microbial communities is critical when developing an effective product that works in the rhizosphere (edaphic section under the influence of the root, where numerous processes promoted by microorganisms take place). Many rhizosphere-targeted products incorporating microbial consortia, for example, generate very inconsistent outcomes, depending on the plant, the habitat, and the soil in which they are used. This is due to the microbial inoculant’s failure to colonize and establish itself in the growth medium in order to serve its purpose.
Because of its enormous diversity, soil appears to be a perfect ecosystem for researching microbial ecology; however, it is also likely the medium that poses the most technical challenges to microbiologists. This is due not only to the magnitude of their microbial community and the vast number of species present, but also to the medium’s multiphasic character and the fact that only 99% of the microorganisms in these microbial communities can be isolated and cultivated on standard cultivation mediums. This is why molecular approaches to microbial community research are so important. Environmental genomics, also known as metagenomics, entails the extraction and sequencing of all DNA from the microbial community being studied. By studying these genes we can learn how these microbial communities operate as well as their phylogenetic composition. Massive sequencing techniques enable us to analyze measurable changes in response to environmental variations, both naturally occurring and induced by the application of a microbiological product, allowing us to gain insight into all of the soil’s hidden diversity. In this way, at the MAAVi Innovation Center (in collaboration with Biome-makers), we conduct microbial ecology studies in order to develop microbial consortia for crop- and soil-directed applications, as well as analyze whether the microbial consortium is capable of colonizing the soil once introduced, and what physicochemical or microbiological characteristics of the soil have been decisive for this colonization.
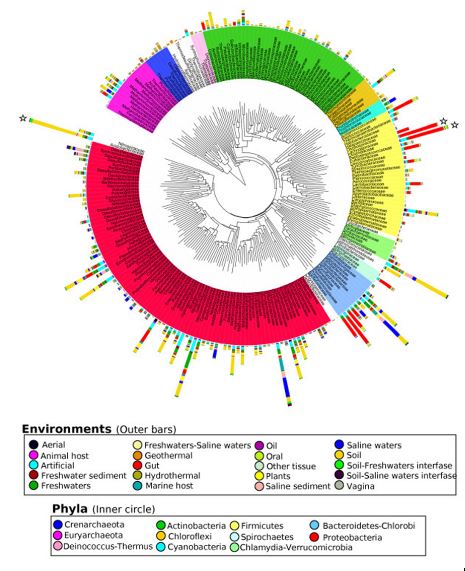
Universal phylogenetic marker
The sequencing of the 16S rRNA, a tiny subunit ribosomal gene that provides information about the kinds of microorganisms present in a specific habitat, is a major factor in determining the extent of this hidden diversity. One of the key areas of research over the past 30 years has been the study of this gene, which has changed how microbial ecology is conducted. We are able to determine evolutionary relationships from the ribosomal gene sequences because:
- They are essential for the synthesis of proteins and are found in all organisms, frequently with multiple copies.
- The protein’s primary and secondary structures, which contain both variable and other highly conserved regions, make it possible it to design both broad-spectrum and specialized primers.
- Because their sequences have stayed remarkably consistent throughout evolution and because changes to their sequence appear to happen very slowly, they can be thought of as molecular clocks.
These traits help us to recognize phylogenetic variants and identify shared evolutionary origins and developmental patterns. Conceptually, random tree structures are simple. 16S rRNA sequences from various species are compared to one another, and any variations are evaluated and used as a gauge of how far apart they evolved from one another.
*Tamames et al. BMC MICROBIOLOGÍA 2010, 10:85. Environmental distribution of prokaryotic taxa
Phages
Bacteriophages, also known as phages for short (from the Greek phagein, which means “to eat”), are the most prevalent type of life on Earth and bacteria’s primary enemies. They are 100 times smaller than bacteria and are in charge of controlling harmful microorganisms. Every 48 hours, they replace half of the world’s bacterial population; the human gut alone contains one trillion phages, but they can also be found on the skin, in food, and crops.
Bacteriophages are naturally occurring microorganisms that are capable of infecting and killing bacteria. Discover how they behave.
The most common mode of action of phages against bacteria is bacteriolysis, which occurs at the end of the phage lytic cycle. (Figure).
In order to reproduce, phages must first enter the host bacterial cell. Cells with a cell wall, like most bacteria, are infected differently than animal cells without a cell wall. As a result, the phages adhere to particular receptors on the exterior of the bacterial cell, creating a hole in the bacterial membrane through which they inject their genetic material. The phage DNA self-replicates inside the infected bacterium and is then integrated into bacterial infectious particles produced from chemicals isolated from the bacterial host. The formation and release of phage particles allows the phage to attack more bacteria in an exponential pattern, resulting in a faster killing impact than other alternatives.
There are several advantages to using phages as bacterial biocontrol agents. They are bacterium-specific, so other strains similar to the pathogenic strain are unaffected, and they have fast mutation rates, enabling them to adapt quickly to any resistance. MAAVi develops and formulates phages on a big scale to combat various bacterial diseases such as those produced by the pathogen Erwinia amylovora.
Scholars believe that RNA is the initial hereditary material, arguing that DNA would have replaced it relatively quickly because it is a more stable molecule than RNA.
Hence, the Last Universal Common Ancestor (LUCA) of the three domains of life would have been a cell with DNA, according to this theory. But how did LUCA get its DNA?
The conversion of cellular genomes from RNA to DNA requires lysogeny by a DNA “founder virus”, followed by host gene migration into the DNA genome. (b) It is believed that three founder viruses, fvB, fvA, and fvE, infected the earliest forms of Bacteria, Archaea, and Eukarya, respectively. It is worth noting that the fvA and fvE viruses are more closely related than the fvB virus. As a result of viral infection, the genomes of these three ancestral lineages
would have eventually evolved from RNA to DNA. Other cell lines derived from the Last Universal Common Ancestor (LUCA) that retained the RNA genome would have presumably died out.
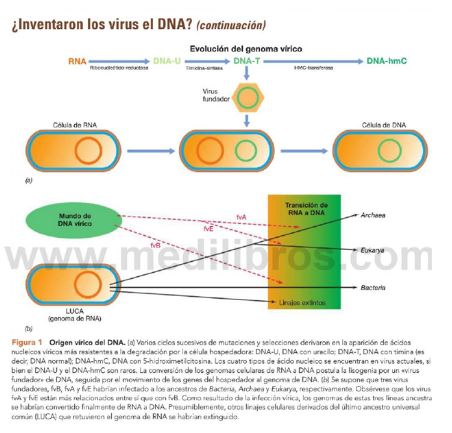
In the area of symbionts, we co-culture symbiotic endophytic microorganisms with their host plant species, both in vitro and on a large scale. This one-of-a-kind system mimics how symbionts interact in nature and has two advantages: i) it enables us to mass-produce symbionts that support plant development; and ii) it allows us to study the molecules produced by the molecular interaction established between the two species. Many of these molecules have varied effects on the plant, such as biostimulation and defense activation, and may be useful in different projects.
Curiosities: How can fungi aid in the battle against climate change? Read all about it
Arbuscular mycorrhizal fungi as carbon sinks
Arbuscular mycorrhizal fungi (AMF) serve an essential role in carbon accumulation by producing glomalin, a glycoprotein that contributes to the stability of soil aggregates. Glomalin has a half-life of 6 to 42 years, resulting in a sluggish rate of decay, making them effective carbon sinks.
Steps in the development of arbuscular mycorrhizae.
Plant roots produce strigolactones, which promote spore germination and hyphal growth as well as fungal spore and hyphal physiological activity.
Myc factors are molecules produced by fungi that are functionally characterized by their capacity to induce calcium influxes into root epidermal cells and activate plant symbiosis genes.
We are able to collect all of these essential symbiotic molecules in our co-culture system.
When AM fungi reach the root, they create hyphopodia, which are appressoria formed by adult hyphae rather than germ tubes.
Plant cells generate a prepenetration apparatus assembly (PPA) as a result of successive chemical and mechanical stimulus. Following that, a fungal hypha extending from the hyphopodium penetrates the PPA, guiding the fungi through the root cells and into the topmost layer.
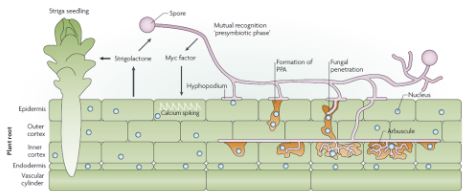
Research Areas
The natural way to overcome diseases
Plant diseases have traditionally been tackled by the widespread use of biocidal agents, which have been shown to have negative impacts on both the environment and the health of humans and many other living species, such as insects. Their population has been significantly diminished on a global scale owing to the misuse of these hazardous compounds, particularly bees, who are required to pollinate thousands of crops throughout the world.
At Kimitec, we have an area in charge of identifying and evaluating different candidates obtained from nature against plant pathogens such as fungi or nematodes, in order to find natural solutions with the same efficiency as synthetic chemistry, to combat the diseases that these cause on crops for farmers all over the world.
Phytopathology is the study of plant diseases. It analyzes the infectious organisms that can cause them, such as fungi, viruses, bacteria, and nematodes, as well as the abiotic factors, with the exception of pest insects researched in entomology.
We fine-tune and conduct bioassays with multiple pathogens, mostly fungi and nematodes, and analyze them using our Hit 2 Lead approach.
We also carry out diagnostic procedures to identify diseases observed in the field during trials or affecting producers with whom we collaborate.
Would you like to learn more about bioassays and diagnostic procedures?
BIOASSAYS
We perform bioassays to assess the efficacy of different candidates:
- In the first phase, in vitro tests are performed to assess spore germination and mycelial growth index in the case of fungi, or mortality in the case of nematodes, in order to do a preliminary screening that allows us to exclude inactive candidates.
- In a second phase, in vitro tests using plant material are performed with those candidates which have demonstrated efficacy or, in the case of obligate biotrophs, need host organisms to develop. In this phase, we use an approach that mimics natural conditions, allowing us to continue excluding candidates with poor efficacy until we reach our LEADS.
- Finally, at MAAVi, studies are conducted in “semifield” conditions in controlled environment greenhouses to validate the efficacy achieved in the previous phases
DIAGNOSTIC PROCEDURE
The cornerstone of disease management in plants is diagnosis. A correct diagnosis is important to make the appropriate decisions to combat diseases and achieve successful control. A misdiagnosis may result in serious economic losses for producers.
At the MAAVi facilities, we have a laboratory equipped to diagnose all types of crops affected by different diseases from across the world. This allows us to detect various disease-causing agents such as viruses, bacteria, fungi, oomycetes, nematodes, and so on.
Research Areas
Molecular Biology
Molecular biology studies the structure, function, and composition of biologically important molecules in order to understand the processes that occur in organisms. These molecules are mostly nucleic acids (DNA and RNA), which contain an organism’s genetic information, and proteins, which perform vital functions for that organism (structural, transport, defense, and so on).
The study of these molecules has made significant progress with the introduction of techniques like massive sequencing, which allows us to evaluate the nucleic acids contained in a cell, tissue, organ, or complete organism in depth and on a large scale.
The advancement of molecular biology has also enabled the development of disruptive technologies such as RNA interference, which is based on the discovery that earned Andrew Z. Fire, Craig and C. Mello the Nobel Prize in Medicine and Physiology in 2006.
All living beings’ genetic information is stored in nucleic acids, which are formed by the union of distinct “pieces” known as nucleotides (equivalent to the letters of an alphabet). Sequencing allows us to decode these letters in order to understand the genetic information of every living organism.
Massive sequencing, or NGS for its English acronym “Nest Generation Sequencing,” comprises all types of large-scale and parallel nucleic acid sequencing, considered the most advanced genetic research technique available today.
Do you want to know more about the sequencing techniques that we carry out at MAAVi?
With massive sequencing, we may discover something as basic about an organism as its genome (the whole of its DNA), or its transcriptome (the collection of all RNA molecules). Additionally, the distribution of bacteria and fungi in an environmental sample can also be precisely mapped through metabarcoding, and their genomes can be reconstructed through metagenomics.
At MAAVi, we gather all of this molecular data using the Illumina NovaSeq 6000 system, the most reliable, scalable, and comprehensive sequencer on the market, which produces up to 6 TB of data per study and enables us to obtain accurate and comprehensive results.
A significant computational advancement is needed to analyze the vast quantity of data generated by the massive sequencing studies. The use of bioinformatics is essential for this purpose.
RNA interference natural
NA interference (or RNAi) or post-transcriptional gene silencing is a universal biological response to double-stranded RNA (dsRNA) molecules that affects gene expression and protein coding. RNAi is currently employed as a tool determine the function of any gene, but it has made significant progress as an emerging technology for the design of new drugs. MAAVi collaborates with Sylentis, a PharmaMar group company that is a pioneer in the development of innovative medicines based on RNA interference (RNAi) technology, to carry out the rational development of new plant protection products.
Would you like to learn more about RNA interference?
RNAi pathway triggers include viral-derived double-stranded RNA, abnormal transcription of repetitive regions in the genome such as transposons, and pre-microRNAs. A simple model of the RNAi pathway consists of two phases: the first, in which the triggering double-stranded RNA is processed by RNase II Dicer enzymes into short double-stranded small interfering RNA (siRNA). In the second phase, the generated siRNAs are loaded into a molecular complex called RISC (RNA-induced silencing complex) and a single-stranded RNA is created that hybridizes with the target messenger RNA, inducing gene silencing. This gene silencing is the result of nucleotide degradation of messenger RNA by the enzyme RNase H Argonaut.
This natural mechanism can be experimentally triggered by the exogenous introduction of long double-stranded RNAs (dsRNAs) or siRNAs, typically between 20 and 24 nucleotides, with the goal of not only conducting functional genomics studies, but also developing therapies for the pharmacological treatment of viral infections and even many types of cancer. At MAAVi, we use this technology to develop biopesticides and bioherbicides.
Research Areas
Bioinformatics
The field of bioinformatics focuses on the research and development of statistical and computational tools for the analysis and use of biological data. These tools use computational resources to solve biological problems that extend beyond the limits of human understanding, such as the extraction of information from biological data produced on a large scale by massive sequencing. Bioinformatics is especially helpful for comparing DNA, RNA, and protein sequences both within and across species in order to study the evolutionary connections between organisms and try to ascribe functions to particular genes and proteins. In that sense, bioinformatics is the linguistics of molecular biology, analyzing patterns in DNA, RNA, and protein sequences in the same manner that linguistics examines patterns in language.
Would you like to know more about the use of bioinformatics at MAAVi?
MAAVi’s bioinformatics area is in charge of performing a variety of analyses, including the alignment of DNA, RNA, and protein sequences, protein structure prediction, computation of gene ontologies, and the search for evolutionary links between different organisms. However, its biggest influence is seen in the large scale analysis of biological data from massive sequencing, highlighting the alignment and annotation of genomes, the ab initio prediction of genes and the computation of differential expression to understand how the genes of different species are regulated. To do this, we employ programming languages like Python and R, as well as high-performance computing on computer clusters using the UNIX operating system.
Research Areas
Artificial Intelligence
The objective of Artificial Intelligence is to develop intelligent machines, particularly computer programs, capable of performing complicated tasks akin to those performed by humans, such as reasoning or learning. Nowadays, artificial intelligence is able to play chess, communicate concepts through drawings, operate self-driving vehicles, and even write stories.
Artificial Intelligence is capable of detecting patterns that are not evident to humans with remarkable precision, thanks to the performance of routine tasks in which large amounts of data are analyzed reliably and without getting tired. MAAVi’s artificial intelligence platform incorporates machine learning and deep learning.
Both machine learning and deep learning are Artificial Intelligence subfields that differ in how they do automated learning. In this way, machine learning allows the computer to learn on its own by providing a set of rules to an algorithm, which it applies when relevant data are found. As a result, it is capable of adjusting existing rules and developing new ones in order to increase its success rate.
Deep learning, on the other hand, varies from machine learning in that it incorporates more complicated and detailed automated learning. Deep learning algorithms are built on artificial neural networks, also known as simulated neural networks, which imitate how the human brain operates.
At MAAVi, we use both machine learning and deep learning, integrating regression, random forests, dimensionality reduction, and unsupervised clustering models for tasks like characterizing an organism’s transcriptomic response to the application of a candidate, or exploring the chemical space of any given candidate. In addition, graph neural networks and encoders-decoders are coupled with these machine learning models.
Research Areas
Phytotrons LINNA
We have 6 growth chambers, or phytotrons, in our LINNA platform where plants can be grown under controlled conditions. They are used for both seed-grown and in vitro plants. We set up each of these growth chambers with the ideal conditions for the target crop under study. We may also recreate varying or extreme weather conditions that are characteristic of specific regions in order to understand how diverse weather conditions around the world impact crop growth and development. For instance, heat stress, water stress, low light, etc. All of this is dependent on the specifics of each trial, which are critical in the development of biostimulants, bioherbicides, and biopesticides.
Would you like to learn more about our controlled conditions growth chambers?
Under controlled growth conditions, several factors are configured, monitored, and recorded in real time and historical data. We may adjust the photoperiod length, humidity, CO2 concentration, and temperature. Each phytotron is 24 m2 in size and can hold more than 2,000 plants. They are fully adaptable, where the height between shelves may be adjusted to different layouts to carry out varied tests, ranging from herbaceous to taller plants.
Would you like to know which conditions we can simulate in our phytotrons?
The temperature range is 5 °C to above 40 °C. The range of humidity is 10% to 95%. It is possible to maintain CO2 levels between 100 ppm and 3,000 ppm.
Research Areas
Trial area
Our solutions are tested in the “lungs” of the MAAVi Innovation Center.
The analysis of the behavior of our solutions under greenhouse testing conditions is one of the key elements in our research process. MAAVi has green lungs, check it out
At MAAVi, we conduct “in planta” testing in boxes and growth chambers where we have complete control over the growth conditions. This not only enables us to organize testing throughout the year, but also to know how our solutions will perform under realistic climate conditions.
- We germinate and grow healthy plants in two seedbed sections and a healthy plant chamber.
- We have 30 boxes for testing biopesticides, probiotics, and biostimulants in addition to 5 boxes for disease assessment.
- 12 growth chambers or phytotrons.
With all the facilities combined, we can accommodate more than 20,000 plants in total. Do you wish to learn how they are distributed? Keep reading!
Healthy plants Chamber
This 86 m2 unit has temperature, humidity, and photoperiod control systems, allowing for year-round plant growth. LED lighting produce red and blue wavelengths that are essential for photosynthesis and plant development. These facilities allow us to produce a large number of healthy, high-quality plants that are free of pathogens and bacteria. The chamber includes 60 shelves spread at four levels of height, allowing for a capacity of more than 3,000 plants and a rotation of more than 1,200 plants every two weeks.
Plants are sent from this unit to the Pests, Phytopathology, and Semi-field departments for formulation validation testing, as well as the Entomology department for use as insect meal. This chamber provides the main horticultural crops to the various units, which include beans, tomatoes, peppers, eggplants, cucumbers, and zucchini. However, we also grow agronomically important plants from other regions, such as tobacco, cotton, soybeans, and sunflowers.
Semi-field area
MAAVi has more than 3,100 m2 of facilities organized into several units to respond to the rising demand for testing and thereby be able to respond to the present agricultural difficulties.
- Seedbeds for the year-round planting and development of healthy, high-quality plants.
- Greenhouses for testing biostimulants and probiotics.
- 35 boxes (20–25 m2) for research on biopesticides and the assessment of formulations’ efficacy against various pests of agronomic importance. They all have special features that allow working in environments free of external or cross contamination.
We have state-of-the-art facilities equipped with cutting-edge technological equipment that allow us to get the most out of our research. As a result, the greenhouses are outfitted with shading meshes that allow us to reduce the temperature inside, evaporative cooling panel systems that allow us to regulate the temperature and humidity of the compartments, greenhouses with lateral and overhead ventilation, irrigation by nebulizers or floodable tables, and anti-insect meshes that provide advanced control of thrips and insects of similar size.
Phytotrons
We have 12 growth chambers, or phytotrons, where plants can be grown under controlled conditions. They are used for both seed-grown and in vitro plants. We set up each of these growth chambers with the ideal conditions for the target crop under study. We may also recreate varying or extreme weather conditions that are characteristic of specific regions in order to understand how diverse weather conditions around the world impact crop growth and development. For instance, heat stress, water stress, low light, etc. All of this is dependent on the specifics of each trial, which are critical in the development of biostimulants, bioherbicides, and biopesticides.
Under controlled growth conditions, several factors are configured, monitored, and recorded in real time and historical data. We may adjust the photoperiod length, humidity, CO2 concentration, and temperature.
Our AI or LINNA area comprises six full growth chambers, each of which is 24 m2 in size and can hold more than 2,000 plants. They are fully adaptable, where the height between shelves may be adjusted to different layouts to carry out varied tests, ranging from herbaceous to taller plants. The temperature range is 5 °C to above 40 °C. The range of humidity is 10% to 95%. It is possible to maintain CO2 levels between 100 ppm and 3,000 ppm.
We have six phytotrons more distributed by work areas:
- A cutting-edge walk-in phytotron that allows for exact control of radiation, temperature, relative humidity, and CO2 levels, which supports growth of both small and tall plants.
- A walk-in chamber equipped to operate with high radiation and a large number of plants, allowing us to work under varying conditions to achieve specific goals.
- Three PLH-LED phytotrons with specialized LED tubes for plant growth, AR12 spectrum.
- A PLH-LED phytotron powered by red and blue LEDs. T
All of these chambers are software-controlled, allowing us to monitor a wide variety of parameters needed for scientific trials.
The combined capacity of these six units is 1,000 plants.
Research Areas
Hydrolysis
At Kimitec, hydrolysis technology has become one of the most important sources of biostimulants for crops
Hydrolysis is the process of breaking down large molecules into smaller components that may then be metabolized by other organisms. Protein hydrolysis is widely employed in agriculture to extract free amino acids or short chains of these, known as oligopeptides, which have powerful biostimulant properties.
There are three forms of hydrolysis: chemical hydrolysis, which utilizes strong acids to aggressively break down proteins, generating compounds not present in nature and yielding substantial concentrations of salts due to the use of powerful alkalis to neutralize the acids. The products that are obtained in this manner are not permitted in organic agriculture.
There are two approaches to carry out hydrolysis in a natural and respectful manner: those based on guided enzymatic attacks and those based on microbial fermentations.
Enzymatic hydrolysis produces amino acid and oligopeptide combinations known as protein hydrolysates, which have several uses in agriculture, aquaculture, livestock farming, the food industry, and cosmetics. Their composition will be determined mostly by the origin of the protein and the enzyme or enzyme cocktail utilized.
Microbial hydrolysis produces more complex compounds because microorganisms have an extensive arsenal of enzymes capable of hydrolyzing many more components of the natural matrix as well as secreting new molecules.
Enzymatic processes are chemical reactions catalyzed by an enzyme that uses water to break down a molecule into two or more fractions. When the enzyme is applied to a solution containing proteins, these are broken down into smaller chains of amino acids known as hydrolyzed proteins. Due to the specificity and selectivity of enzymes, the process variables may be adjusted to produce the appropriate type of hydrolysate. The size of the peptide chains generated, as well as the quality and uniformity of the final product, depend on the process variables (temperature, pH), identification of the enzyme/substrate combination, and the appropriate ratio. To achieve a good reaction rate and maximize the enzymatic efficiency, it is critical to understand all the reaction variables in an enzyme hydrolysis process.
One of the most important benefits of non-chemical hydrolytic processes is the low frequency of amino acids in the racemic form D, which cannot be taken by plants; yet, this form is far more common in chemical processes.
The microbial hydrolysis or fermentation process is slower and more expensive, but it produces a higher quality product because the presence of L-form amino acids is the highest of the three options, and during the microbial growth process, in addition to hydrolysis reactions, biosynthesis processes give rise to molecules with higher biostimulant activity, such as polyamines. Microbial fermentation processes are often carried out with bacteria of the Bacillus or Aspergillus species, which have a diverse set of enzymes capable of breaking down a large number of plant compounds while also creating molecules with specific biostimulant activity.